A decade ago, the Nobel Prize in Chemistry was awarded to a trio of researchers for the development of super-resolved fluorescence microscopy. The announcement at the time stated that the researchers’ work had “brought optical microscopy into the nanodimension.” Indeed, their work broke the diffraction-limited resolution barrier. In conventional light microscopy, the diffraction limit, or the physical limit for the maximum resolution, is 200 nm.
One of the two techniques recognized by the prize was single-molecule localization microscopy (SMLM). Actually, SMLM is a group of techniques. It includes stochastic optical reconstruction microscopy (STORM), DNA-based point accumulation for imaging in nanoscale topography (DNA-PAINT), and photoactivated localization microscopy (PALM). The three approaches vary in how they carry out the random activation of a subset of fluorescent molecules—the cornerstone of SMLM. The data analysis leads to the resolution of structures at the nanoscale level.
SMLM was developed by two of the Nobel Prize winners, Eric Betzig, PhD, and William E. Moerner, PhD. Betzig is a senior fellow at the Janelia Research Campus, a professor of molecular and cell biology and a Howard Hughes Medical Institute Investigator at the University of California, Berkeley. Moerner is a professor of chemistry and a professor of applied physics at Stanford University.
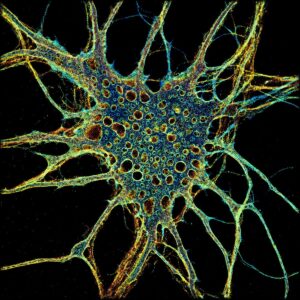
Also recognized in 2014 was stimulated emission depletion (STED) microscopy, invented by Stefan W. Hell, PhD, director of both the Max Planck Institute for Multidisciplinary Sciences in Göttingen and the Max Planck Institute for Medical Research in Heidelberg.
The discoveries instigated progress on two fronts. On the scientific front, it became possible to visualize molecules (and the pathways of individual molecules) inside living cells. On the technological front, it became possible to develop commercial nanoscopy instruments. Developments on each of these fronts are reinforcing each other. Technology providers are introducing increasingly accessible microscopy systems, removing the need for highly expert users, and bringing high-powered microscopy to more and more biologists. And a growing community of users is encouraging more commercial activity.
Between the years 2016 and 2017, several companies launched or expanded into this space. Here, GEN spoke with some of these players to learn how they are making super-resolution microscopy easier, cheaper, and more accessible than ever.
ONI’s black box breaks barriers
When the Nobel Prize in Chemistry was awarded in 2014, STORM started enjoying wider recognition. But according to Jason Jell, PhD, vice president of marketing at ONI, the technology “wasn’t particularly accessible to anyone.” Users needed to be highly specialized, with expertise in physics, chemistry, and biology. In 2016, ONI was started to bring the technology to researchers who work in traditional laboratories and aren’t microscopy experts.
ONI was spun out of academic research led by Bo Jing, DPhil, at Oxford University. Besides founding ONI, Jing served as the company’s CEO until he left to pursue other interests in 2023. ONI’s current CEO is Paul Scagnetti, PhD, formerly Illumina’s vice president of corporate and business development. Now, at about 100 people strong, the company remains in the United Kingdom but has recently opened a U.S. office in San Diego.
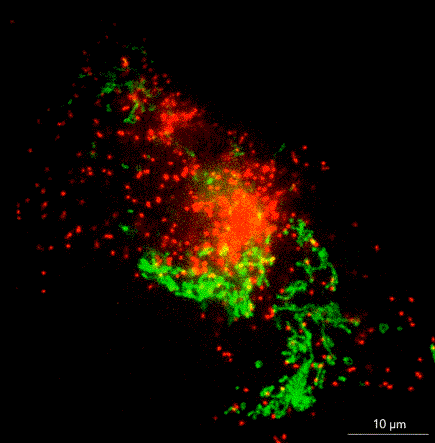
What does it take to bring SMLM to non-microscopists? Jell suggests removing barriers such as the need for a darkroom, temperature control, a special table, or a technician to tweak or align the instrument. In ONI’s instrument, the nanoimager, a microscope floats within an enclosure with stable optics that are easy to maintain. The company has swapped out commercially available lasers for ones that it has designed in house, and it has built flow cells to allow multiple lanes on a single chip.
Jell suggests that SMLM will push microscopy to overcome assumptions. For example, that microscopy is only qualitative. SMLM doesn’t just produce images. It also generates data. In a sense, the images are data. The rows and rows of points that constitute an image are actually coordinate-based datasets that can be used not only to pinpoint the 3D position of molecules, but also to determine how proteins interact.
Microscopy has also driven assumptions about biology, notes Jell, based on the resolutions that could be captured using the microscopes that have been available to date. But techniques like SMLM offer a new granularity and allow researchers to change their fundamental thinking of how organelles are arranged and how molecular structures are set up.
Une entreprise de microscopes
Many researchers already have microscopes. And many of those scientists are already doing fluorescence microscopy. But they want better resolution because they are tired of hitting the “diffraction barrier” and being unable to see anything smaller than 200 nm.
This limitation is especially vexing when signals from individual fluorophores interfere with each other. To distinguish between closely packed fluorophores, researchers may resort to a temporal separation technique such as STORM, where fluorophores are stochastically activated. Different fluorophores emit light at different times so that signals do not overlap. Such techniques can involve a lot of repetition, with 1,000 to 100,000 frames being needed to reconstruct an image at nanoscale.
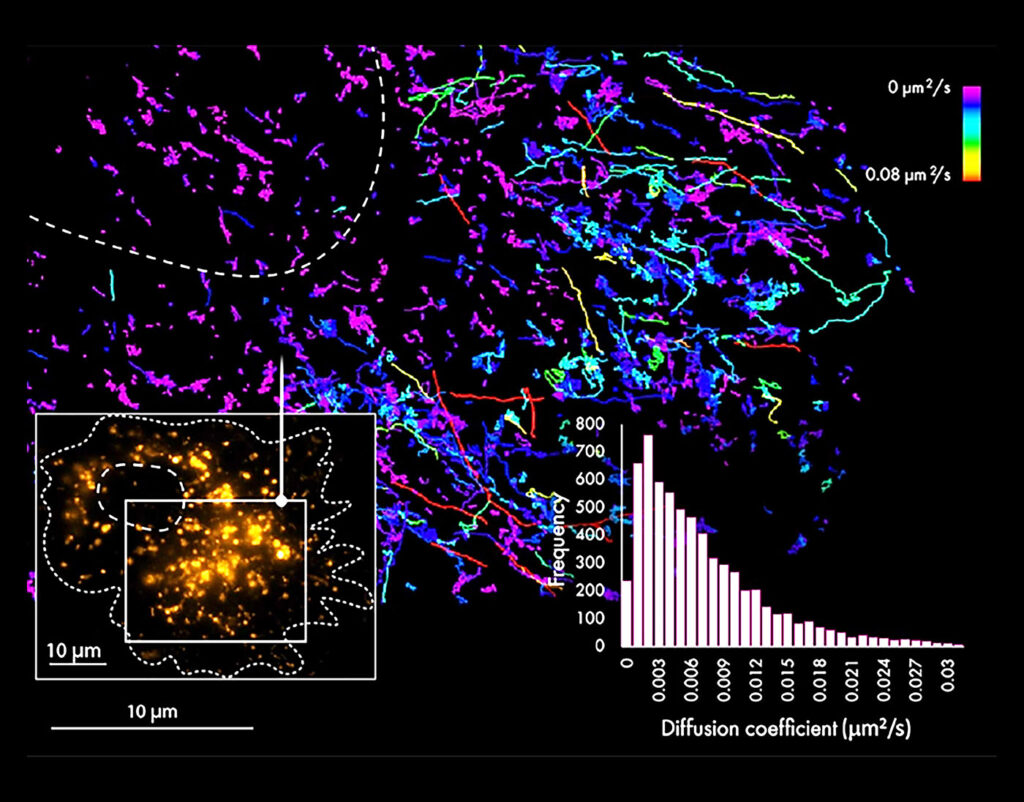
To help scientists overcome the diffraction barrier, the French company Abbelight offers a solution: SAFe, a multimodal imaging platform that is designed to fit with the camera port of any inverted microscope. Two of the available SAFe configurations support SMLM, accommodating researchers who want to break the diffraction barrier and prefer to do so by upgrading their existing microscope as opposed to replacing it. Essentially, these configurations incorporate super-resolution add-ons that can transform a microscope into a fluorescence super-resolution microscope.
Abbelight’s technology is based on optical engineering research that was conducted at Paris-Saclay University by Nicolas Bourg, PhD, when he was earning his doctorate. He co-founded Abbelight in 2016 and has since served the company as CTO.
According to Bourg, a super-resolution journey needn’t be difficult, especially since Abbelight can help with the journey’s most difficult part—getting started. Also, Abbelight understands that it may need to accommodate different itineraries as users’ priorities change. According to Bourg, users were focused on resolution. But now, they’re beginning to pay more attention to ease of use, reliability, robustness, and the ability to image several biological structures at nanoscale.
Bourg points out that the key for SMLM applications in biology is the ability to obtain an image of one protein and to correlate that image with one of another protein. He adds that an image of just one structure is great, but that it is useless unless you can compare it to another one.
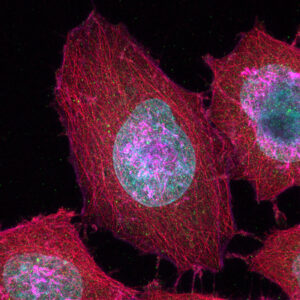
Some researchers prefer a full microscope. To accommodate these researchers, Abbelight typically works with Evident (a former Olympus subsidiary that became an independent corporation in 2022). Evident has been in the super-resolution space since 2017. In addition to working with Abbelight, Evident has its own SpinSR system, which utilizes the SoRA spinning disk confocal system from Yokogawa Life Sciences to enable super-resolution imaging down to 120 nm. Evident also has the Fluoview point scanning confocal system, which can image down to 110 nm using super-resolution algorithms.
Academics show a lot of interest in moving into SMLM, Bourg notes. However, Abbelight is also working with biotechnology companies and with pharmaceutical companies such as Sanofi.
Bruker joins the SMLM mix
In 2014, back when the pioneers of SMLM were receiving their Nobel prize, Bruker acquired Vutara—a University of Utah spin-off company that had developed an SMLM microscope. Vutara’s microscope was added to Bruker’s existing portfolio, which already included confocal fluorescence and atomic force microscopes.
Vutara’s microscope also had capabilities for acquiring 3D Z-stack images of tissue samples. Indeed, it incorporated bi-plane technology developed in the laboratory of Joerg Bewersdorf, PhD, professor of cell biology, biomedical engineering, and physics at Yale School of Medicine.
In 2020, Bruker released an improved version of the microscope, the Vutara VXL. This instrument supports multiplexing applications such as DNA-PAINT and spatial multiomics imaging.
Bruker’s Vutara super-resolution microscopy product manager, Winfried Wiegraebe, PhD, says that SMLM technology currently appeals to cell biologists and neuroscientists. The former are most interested in using super-resolution technology to understand the structures and interactions of subcellular organelles. The latter are most interested in using the technology to study synaptic and cytoskeletal structures.
Wiegraebe notes that multiomics applications are another area where localization microscopy is used. Chromatin tracing applications explore the 3D structure of the chromatin in individual cells, and smFISH-based imaging explores the spatial relationship between subcellular structures within specialized cells such as myocytes.
SMLM experts peer into the future
Bourg thinks that SMLM will expand the role that microscopy plays in drug discovery. In addition, he predicts that SMLM will improve nanoparticle characterization, both externally (showing particle shapes) and internally (showing particle contents). Extracellular vesicles are a rapidly growing field and, Bourg says, SMLM is “perfect for them.” Currently, the characterization of extracellular vesicles and lipid nanoparticles is done with electron microscopy, but when it is done with SMLM, the addition of fluorescence information enables a more targeted approach. The next big challenge, Bourg observes, will be SMLM in living cells.
The market for super-resolution systems is still in its infancy even though it is based on technologies that were developed roughly 20 years. For example, SMLM emerged in 2006, and STED microscopy goes back to the mid-90s. Nonetheless, with the technological improvements that have been made over the past 10 years, the market is poised for robust growth. According to Future Market Insights, the market is growing at an annual rate of 10.2% and will reach a value of $9.5 billion by 2033.
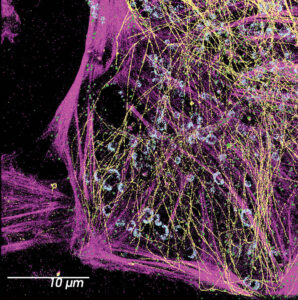
Avi Smith, Associate Product Manager at Evident, notes that as super-resolution microscopy becomes more accessible, users are showing more interest in multimodal systems, such as systems that combine confocal laser-scanning microscopy and SMLM. With these systems, imaging data between modalities can be correlated. The company also sees the integration of super-resolution imaging into high-content screening systems to automate the analysis and quantification of results.
Wiegraebe anticipates that “scientists will discover the power of combining omics and super-resolution approaches.” He also expects a range of improvements in SMLM: DNA-PAINT-based technologies will be extended by techniques such as resolution enhancement by sequential imaging; multiplexed labeling will dramatically increase the number of targets imaged in one sample; and improved photoswitchable proteins and live cell-compatible organic dyes will improve live cell imaging.