Voltmeters are routinely used to measure the voltages in electrical circuits and across batteries. But imagine a voltmeter so small that it can slip into a living cell and measure the voltage across the outer layer of structures known as organelles. That’s what a team of researchers at the University of Chicago has developed, as reported in a recent paper in Nature Nanotechnology.
Many biological processes, including the beating of the heart and the transmission of pain signals along nerves, rely on the flow of electricity. That in turn requires electric potentials, or voltages, across the plasma membranes that enclose cells and organelles. Scientists have long known such membrane potentials exist, but those across organelles were too small to measure, hiding them from view.
“Many scientists thought some organelles didn’t have this membrane potential,” says Yamuna Krishnan, a professor of chemistry at the University of Chicago.
To reconcile the issue, Krishnan, along with graduate student Anand Saminathan and their colleagues at the University of Chicago, developed a nano-scale voltmeter and used it to measure the membrane potentials of various organelles. The device, called Voltair, has two components: a core consisting of two strands of DNA to which two fluorescent molecules are attached. The DNA serves as a targeting mechanism, helping the voltmeter to enter the cells and reach the proper organelle. The two fluorescent molecules act as the ‘probes’ of the voltmeter.
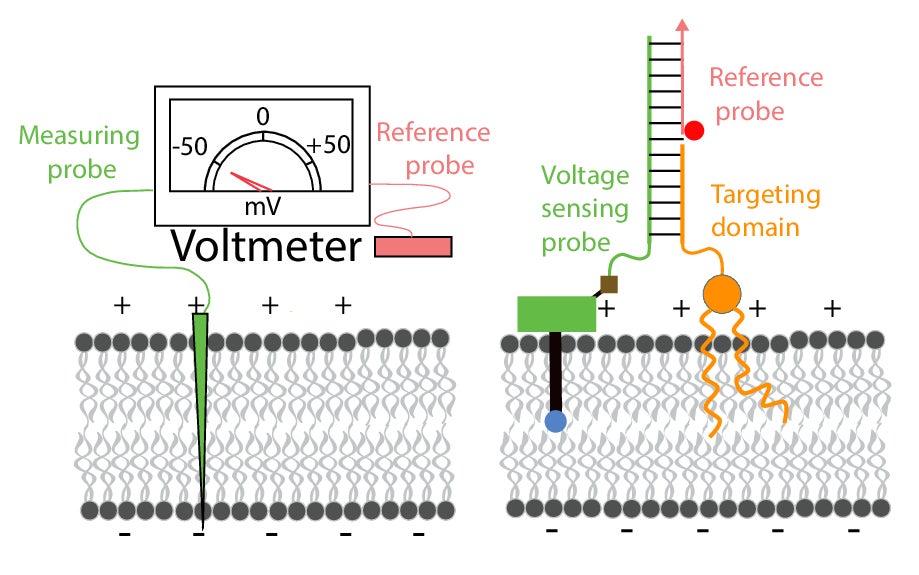
At the organelle, the voltmeter’s reference probe remains outside the membrane while the other one embeds into the membrane. The reference probe has a constant fluorescence intensity, and the embedded one’s intensity fluctuates with the voltage. By measuring the difference in the intensity of the two probes, the researchers can ascertain the potential difference between them.
“The membrane potential was a black box, and so our sensor will open up unexplored territory in biology,” says Krishnan. Cracking open that box could help biologists explain cellular processes, along with the mechanisms that underpin certain diseases and metabolic disorders. That knowledge could, in turn, drive new directions for drug discovery.
“If you look at all drugs approved by the Food and Drug Administration in the USA, the second most-used protein-based drugs act on ion channels in the plasma membrane,” says Krishnan. “But there are 10 times as many ion channels present on organelles that we cannot yet access. The ability to see the membrane potential opens up the possibility of looking at the functions of ion channels in organelles, opening up avenues for drug discovery.”
To read more about the research, explore the paper in Nature Nanotechnology.
Yamuna Krishnan is currently professor of chemistry at the Grossman Institute of Neuroscience at the University of Chicago. She obtained her PhD from the Indian Institute of Science in Bangalore, and started her independent career in 2005 at the National Centre for Biological Sciences, Bangalore, where she pioneered the deployment of DNA nanodevices for quantitative chemical imaging in living systems. She received the Shanti Swarup Bhatnagar Award for Chemical Sciences and the Infosys Prize for Physical Sciences, and was on Cell’s ‘40 under 40’, scientists who are shaping current and future trends in biology.
Anand Saminathan is a graduate student at the Department of Chemistry, University of Chicago. He obtained his B. Tech in chemical engineering from the Indian Institute of Technology, Guwahati, where he developed an interest in techniques to probe the biochemical and biophysical properties of cell membranes. He developed a DNA-based reporter for the absolute membrane potential difference in organelles and believes that interdisciplinary approaches are the future.