Significant technology development is required to establish the feasibility of a muon collider. Mastering it, says Mark Palmer, offers an alternative path to the next energy frontier.

Why is everyone talking about the muon collider?
The physics landscape has changed. We have not seen signs of new particles above the Higgs-boson mass. Typical limits are now well above 1 TeV based on LHC data, which means we need to look for the new physics that we anticipate at higher energies. The consensus during the recent US Snowmass process was that we should aim for 10 TeV in the centre-of-mass. A muon collider has the feature that its expected wall-plug power scales very favourably as you go to the multi-TeV scale. While significant technology development is required to establish the overall feasibility, performance and cost of such a machine, our current performance estimates make it a very interesting candidate. This motivates an active R&D and design programme to validate this approach.
Why was the US Muon Accelerator Program (MAP) discontinued a decade ago?
MAP was approved in early 2011 to assess the feasibility of the technologies required. By 2014, the community had just discovered the Higgs boson and was focused on pursuing a Higgs factory. Mature concepts based on superconducting (ILC) and normal-conducting (CLIC) linear-collider technologies were at hand, and these approaches envisioned subsequent energy upgrades that would enable the exploration of a new-particle spectrum extending into the TeV scale. Because of the relatively low mass of the Higgs, work was also going into a large circular collider design that would represent minimal technical risk. A muon collider, a concept with much lower overall maturity level and with significantly different operating characteristics, did not appear to provide a timely path to realising the Higgs factory.
The other application of interest involving muon–accelerator technologies was the neutrino factory. However, the field concluded that a long-baseline neutrino experiment based on the “superbeam source” represented the best path forward. In a constrained budget environment, the concepts being pursued by MAP didn’t have sufficient priority and support to continue.
What do we know so far about the feasibility of a muon collider?
As the MAP effort concluded, several key R&D and design efforts were nearing completion and were subsequently published. These included demonstrations of normal-conducting RF cavities in multi-Tesla magnetic fields operating with >50 MV/m accelerating gradients, simulated 6D cooling-channel designs capable of achieving the necessary emittance cooling for collider applications, and a measurement of the cooling process at the international Muon Ionization Cooling Experiment (MICE). While MICE only characterised the performance of a partial cooling cell, the precise measurements provided by its tracking detector system confirmed that the muons behaved consistently with the cooling process as described in the simulation codes that were employed to design the cooling channel for a high-brightness muon source.
Any future collider operating at the energy frontier will have to be supported by a global development team
Another key advance was detailed simulations of the performance of a muon-collider detector in the lead-up to the last European strategy update. These efforts, utilising the beam-induced background samples prepared by MAP, demonstrated that useful physics results could be obtained with reasonable assumptions about the performance of the individual elements of the detector.
How are things going with the International Muon Collider Collaboration (IMCC)?
The IMCC, led by CERN with European funding support from the MuCol project, presently coordinates global activities towards R&D and design. The collaboration’s input has been crucial in developing the technically limited timeline towards a multi-TeV muon collider as outlined in the accelerator R&D roadmap commissioned by the European Laboratory Directors Group. The IMCC is making excellent progress towards a reference design for the muon-collider complex as well as defining a cooling demonstrator. An interim report is currently being prepared. However, current funding levels for the effort correspond to roughly half of the estimated levels required to achieve the technically limited timeline. With the strong support for pursuing an energy-frontier muon collider in the US, it is hoped that a fully global effort will be able to support the effort at levels that much more closely match the requirements of a technically limited timeline.
How does the IMCC relate to the P5 recommendations for reinvigorated muon collider R&D at Fermilab?
Any future collider operating at the energy frontier will have to be supported by a global development team, and the issue of where such a machine can be sited will depend on a complex set of circumstances that we certainly can’t predict now. The fundamental goal is to identify the technology and one or more sites where it can be deployed so that we are able to continue our exploration of the fundamental building blocks and processes in the universe for all humankind. Thus, the current IMCC activities are fully aligned with the aspiration expressed by P5 to explore the option for conducting muon collider R&D in the US and exploring the possibility of Fermilab as a host site for a future machine.
What are the key accelerator challenges to be overcome?
While there are a number of challenging subsystems to engineer, the most novel aspect of the machine remains the ionisation cooling channel. Demonstration of the beam operations of a cooling module at high beam intensity will be necessary to give us confidence that the technology is robust enough for high-energy physics applications. In addition to this absolutely unique subsystem of the muon collider, we require detailed end-to-end simulations of the overall machine performance, detailed engineering conceptual designs for all key components, and successful engineering demonstrations of suitable-scale prototypes for several critical systems. These include the target, the fast-ramping magnet system for the high-energy accelerator stages, the large-aperture collider ring magnets that must be adequately shielded against the decay products of the muon beams, and detector subsystems that can robustly operate in an environment with the beam-induced backgrounds from the muon decays.
And the detector challenges?
Tremendous progress in detector technology has resulted from the design and operation of the LHC detectors. Further progress in obtaining precision physics measurements in very high-occupancy environments as we prepare for the HL-LHC provides confidence for the detector requirements of a muon collider, which will have to deal with similar hit rates. While the details of the occupancy in the detectors for these two types of machine are not identical, the concepts being implemented for better time and spatial segmentation appear quite effective for both.
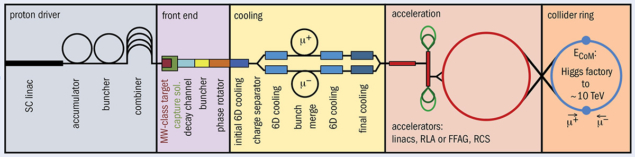
A particular feature of the muon collider detector is the “shielding nozzle” that was first introduced in MAP to protect the innermost detector elements. These nozzles impact the overall physics performance by limiting the near-axis coverage. However, with detailed detector performance studies underway, we are now in a position to carry out detailed detector and shielding studies to optimise these elements for overall physics performance.
How is the vast neutrino flux being addressed?
The very high-energy muon beams in a collider result in a narrow cone of neutrinos being produced in the forward direction as they circulate around the collider ring. When the beams are moving through dipoles, the constant change in transverse direction helps to dilute this flux, but any straight sections in the ring effectively act as a high-energy neutrino source that shines in a specific direction. The tremendous flux of neutrinos from a straight section of a TeV-scale collider are expected to create ionising radiation wherever they exit Earth’s surface. Thus, there are a set of mitigation strategies incorporated into the design effort to make sure that there are absolutely no risks. This includes minimising the number of straight sections, incorporating magnet-movers that allow the vertical trajectories of the beams to be changed slowly throughout the collider, and ensuring that the beams do not exit in populated areas.
What does the timeline for a 10 TeV muon collider look like?
We need to deliver a complete end-to-end reference design in time for the next European strategy update and for the US interim panel review that was recommended in the P5 report. A conceptual design report (CDR) for a demonstrator facility then has to be completed such that construction could begin by around 2030. Over the course of the next decade, the engineering design concepts for each subsystem have to be prepared and prototyping R&D has to be carried out, while also producing a CDR for the high-energy facility, including detailed performance simulations. By the late 2030s, the demonstrator facility and prototyping programme would enable detailed technical specifications for all key systems. Upgrades to the demonstrator facility could be necessary to further clarify performance and technical specifications. The final steps would be to complete a technical design that incorporates results from the demonstrator programme and to develop site-specific plans for the labs that would like to be considered as potential hosts for the facility. The start of 10 TeV collider operations would then be guided by a physics-driven plan, including potential intermediate stages, but likely at least a decade after construction approval.
The current schedule puts physics operations of a high-energy muon collider about five years earlier than an FCC-ee. Is this realistic?
I would characterise these two timelines as being of different types. The FCC-ee timeline is based on an integrated plan for CERN, while the 3 TeV muon collider is explicitly a technically limited plan which assumes that a sufficient funding profile can be provided, and that there are no external constraints that could impact deployment. In other words, the muon-collider timeline remains an aspiration, whereas the FCC-ee timeline attempts to build-in actual deployment constraints.
What is the estimated cost of a 10 TeV muon collider?
At present, the cost estimates rely on broad extrapolations from existing collider systems. While these extrapolations suggest that a multi-TeV muon collider may well be one of the most cost-effective routes to the energy frontier, the uncertainties remain large. To deliver a “realistic” cost estimate, we will require a complete end-to-end reference design, engineering conceptual designs for all of the unique systems required, detailed cost estimates for the engineering conceptual designs and extrapolated cost estimates for the remaining “standard” accelerator systems. With the present technically limited schedule as prepared by the IMCC, this would suggest that a detailed and realistic cost estimate could be available around the end of this decade.
How does a high-energy muon collider fit into the global picture?
There are multiple ways this can fit. At present, we need to acknowledge that the R&D for the magnets for a high-energy proton–proton machine, such as those being pursued in Europe and China, still require an extensive R&D programme. This is likely a multi-decade effort in and of itself, and is commensurate with the timescales needed to carry out muon-collider R&D and design work. Having more than one technology option on the table to achieve our ultimate physics goals is a necessity. Furthermore, the complementarity between lepton- and hadron-collider paths may be needed to support our overarching scientific goals.
A detailed and realistic cost estimate could be available around the end of this decade
From a somewhat different point of view, the potential applications of a high-intensity muon source extend beyond colliders. The technology offers improved performance and new opportunities for other scientific goals such as a high-performance source for future neutrino and charged lepton flavour violation experiments, materials science and active interrogation of complex structures, among others. Clarifying the broader context for the technology is currently being pursued within the IMCC effort.