The growth of advanced fabrication methods, small-scale nanomaterials and the ability to embed flexible circuitry and components into polymeric hosts have given rise to flexible electronics with advanced functionalities. This has become a popular way of building advanced wearable devices in a wide range of industries.
Like any emerging technology, there have been many academic advances, but the flexible and wearable technology space has seen an explosion in commercial growth in recent years. DuPont, for example, has been creating flexible polymer materials for many years. It also offers flexible PCBs.
Despite gaining popularity in recent years, the first flexible electronic device was created back in 1927 in the form of a flexible radio tuner that used a graphite paste. Today’s devices are significantly more advanced. Advances in nanofabrication methods and the ability to integrate nanomaterial-based components (or nanoscale layers of commonly used materials) and circuitry into flexible hosts have been one of the key reasons why flexible electronics have achieved so much commercial growth in recent years.
Solar cells
The development of organic solar cells has created flexible solar cells that can conform to various geometrical surfaces. These have now become a staple solar technology with multiple suppliers, for example, Marlec, Renogy and UKSOL, in the UK.
Flexible solar cells that are also wearable have not been as widely commercialised compared to larger scale flexible solar cells. This is mostly due to market demand, but one company in Sweden, called Peafowl Plasmonics, has created transparent plasmonic-enhanced solar cell devices that can be printed onto polymers and other flexible materials. The company has highlighted the use of the solar cell in watches where the cell wraps around the wearer and provides recharging capabilities for the batteries. Augmented reality/virtual reality glasses have also been touted as a potential application.
A lot of organic solar cells have significantly lower power conversion efficiencies (PCEs) than inorganic solar cells. Peafowl’s technology uses plasmonic nanoparticles in the junction, which absorb more light and provide a resonance among the nanoparticles, which causes the electrons to be collectively excited by each photon. This enables the solar cell to work in low light conditions as well. The company states that “plasmonic nanoparticles can absorb up to 10-times more light than other known materials”. However, no specific PCEs have been publicly mentioned at the time of writing.
While this is an interesting area of flexible/wearable solar cells being commercialised, there is currently a drive to create flexible 2D perovskite solar cells that are deposited on to/integrated into polymer substrates. Bulk perovskites are seen as the future of inorganic solar cells and their PCEs are already almost at the level of commercial silicon systems, yet with a much higher theoretical potential.
The inorganic nature of perovskites means that they can create more efficient solar cells. 2D perovskites currently have challenges with electron cascading, energy carriers relaxing and open-circuit voltage loss, but they are still some way from being commercialised at this stage. If 2D perovskites could eventually mimic the potential of bulk perovskites, and the technical challenges were ironed out, then their efficiencies could far outstrip the PCEs of flexible organic cells.
Graphene in health
The ability to create stretchable electronics, as either e-textiles or polymer-based wearables, has been a great benefit to the health-monitoring space due to the extra comfort and direct interface that a conformal monitoring device offers. Flexible healthcare electronics conform to the wearer and are embedded with sensors and other supporting components (batteries/capacitors and wireless communication technology) to detect changes in the body and/or monitor specific biological levels in a patient, for example, glucose, temperature, or blood oxygen levels.
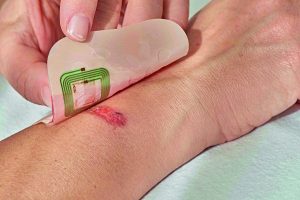
Grapheal has developed a proprietary process to bond graphene on to a biocompatible polymer for a flexible smart medical patch
Many nanomaterials, including carbon nanotubes, silver nanowires and conductive nanoscale polymers have been used in wearable monitoring devices. However, it is graphene that has made a biggest impact because its mechanical properties and inherent flexibility make it an ideal material for deforming with the polymer host and not breaking. Graphene’s electrical conductivity and charge carrier mobility enable very sensitive, small sensors that can be integrated into flexible polymers. The zero-bandgap nature of graphene’s electronic structure can be harnessed to connect the components in the device.
A lot of advances are still academic due to higher regulatory requirements in the medical industry. One company in France, Grapheal, has become a pioneer in this space. Grapheal has created the WoundLAB, which is a wearable and disposable graphene biosensor on a flexible polymer that continuously measures and stores wound-healing data, such as pH, to monitor a patient during the healing process. The medical cloud that is connected to the WoundLAB also provides alerts on infections and moisture levels in the patient and can be accessed via a smartphone app.
When asked why polymers were chosen as the flexible substrate for the smart medical patch, Vincent Bouchiat, CEO of Grapheal, told Electronics Weekly that “our proprietary process enables to strongly bond graphene on to a biocompatible polymer, such as parylene, and it can be used with roll-to-roll synthesis and processing”.
On the use of graphene to ensure that the whole device is flexible, Bouchiat adds: “Graphene is the ideal material for generating bioelectronics sensors and electrodes. Monolayer CVD graphene is super flexible and sturdy and is the ideal material for collecting biological data from vital signs and more complex signals – such as changes in biomarker concentration.”
When asked about his thoughts on the graphene flexible electronics space, Bouchiat says: “We are expanding our pilot line of Graphene-on-Parylene to generate other applications such as RFID gas sensors.” He continues: “I can see more graphene films entering the flexible electronics space in the coming years.”
Graphene has had a key influence on e-textiles, both natural textiles such as cotton, and polymer-based ones including Kevlar. Graphene inks can be printed on to e-textile fibres, or integrated directly into the fibres during production, to create a conductive medium around the body that connects individualised components within the textile. The need for flexible devices in these systems also enables graphene to be a part of the sensing and energy storage devices that provide the health monitoring.
The integration of graphene directly on to/into the fibres enables the excellent conductivity properties of graphene to be harnessed while utilising the natural comfort of the fabric. The high tensile strength and mechanical properties of graphene add an extra layer of durability to the textile as well.
These kinds of e-textiles are mostly still coming through in academic institutions around the world, including the University of Manchester, which is leading a lot of the research via the National Graphene Institute.
There have been commercialisation talks about bringing these e-textiles to market, but for the moment the focus on commercial graphene textiles has centred around thermally regulating textiles rather than e-textiles.
Flexible future
The potential for flexible electronics in wearable devices is greater than ever before due to advances in nanofabrication methods creating ultra-thin layers and the availability of different nanomaterials. Small-scale, conductive and flexible nanomaterials are being integrated into flexible polymers (or deposited on top) to create complex devices that can have a range of functions.