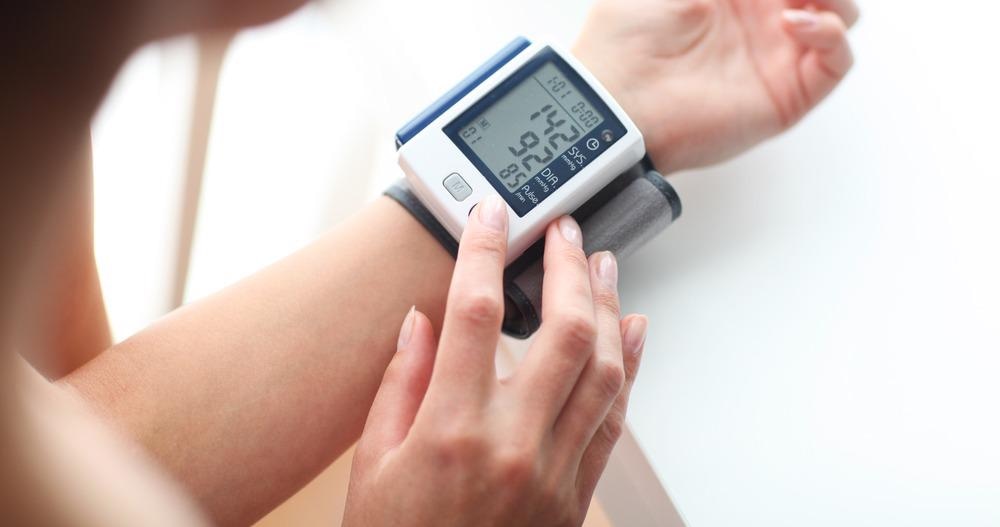
Image Credit: mikeforemniakowski/Shutterstock.com
A group of researchers from the Pennsylvania State University recently developed highly stretchable and flexible microstrip antennas for their future use in sensing devices. In addition to the work published by this group of researchers, an extensive amount of work has been done on advancing the sensing capabilities of stretchable sensors for a wide range of non-invasive health monitoring devices.
An Introduction
The rising costs of healthcare have supported the development of miniature medical devices that can be used by patients remotely while simultaneously abiding by the World Health Organization’s (WHO) requirements for medical devices. These requirements dictate that medical devices should be affordable, sensitive, user-friendly, specific to each health condition, robust, rabid, deliverable, and equipment-free.
Many individuals have already experienced the benefits associated with handheld medical devices. The devices include:
- Electronic sphygmomanometer, which is used to provide patients with blood pressure readings
- Portable electrocardiogram (ECG) monitor
- Glucometers
The handheld technology remains limited in its ability to be worn by the user. Without being wearable, patients are not able to continuously monitor their health parameters throughout the day, which can prevent significant health measurements from being acquired. Taken together, there is a need to incorporate a higher degree of wearability into household medical devices to provide real-time health monitoring systems to patients.
Challenges in Wearable Sensing Devices
Despite the considerable advancements that have been made within the sensors industry, there has been a lack of movement on incorporating these sensors into commercial wearable health monitoring appliances. This status can be attributed to several challenges that sensor developers have faced in the development of these wearable sensing devices.
Some of these challenges include limited comfort of these devices, the presence of several stimuli produced by humans that could interfere with the sensing devices monitoring capabilities, as well as the need to enhance signal delivery and power supply to the sensors within these systems.
Developing Flexible, Stretchable, and Wearable Sensors
Several different mechanical stimulations can be converted into electrical parameters by wearable electromechanical sensors to provide information on the health status of the individual. Mechanical stimulations such as strain, pressure, force, and vibration, can be transduced by various sensing mechanisms, some of which include piezoresistivity, capacitance, and piezoelectricity.
Piezoresistivity, for example, is the most popular transduction mechanism used in the development of stretchable electrochemical sensors. The preference for utilizing this sensing mechanism is largely due to the simple configuration of piezoresisitivity, as well as its excellent sensitivity capabilities and ease of operation.
To further improve the deformation range and gauge factor of a sensor’s piezoresisitivity, several studies have looked at adjusting the contract state of the conductive elements and incorporating a tunneling effect into these sensors.
Capacitance is also widely used for stretchable electrochemical sensors, particularly those aimed towards monitoring various health conditions, as capacitance change can reflect changes in the sensed physical, chemical, or biological stimuli.
An applied load onto capacitive wearable sensors causes a displacement to the plates, which ultimately alters the capacitance values. When incorporated with quadripartite textile electrodes and air-fluorosilicone dielectrics, capacitive sensors have been found to provide a high level of flexibility, sensitivity, robustness, and stability in their ability to measure three-axial forces.
Thirdly, piezoelectricity can be defined as an electromechanical interaction that generates electric charges in certain non-centrosymmetric crystal structure materials following the application of external mechanical stimuli. In addition to its use in several different highly sensitive variation sensors, piezoelectricity has also been investigated for its potential use in the construction of flexible strain/pressure sensors when embedded to flexible polymers.
Stretchable Antennas
In addition to the attempts that have been made to enhance the energy transmission of sensors through various transduction mechanisms, radio frequency (RF) antennas have also been studied for their long-range operational capabilities. One challenge that RF antennas have the potential to address is the limited operating distance of near-field communication (NFC) systems with minimal power consumption.
Wireless sensing stretchable antennas utilize a horn antenna as the interrogator and subsequently measure the reflection from the stretchable sensing antenna. Some of the different stretchable conductors that have been incorporated into these stretchable sensing antennas include various conductive nanofillers such as liquid metal, conductive textiles, and silver nanowires, as well as metalized foams.
Applications of a Stretchable Microstrip Antenna
Since many stretchable conductors can alter the performance capabilities of stretchable antennas, conventional metallic conductors with stretchable structures have also been studied. As compared to the intrinsically stretchable conductive materials that are traditionally used in these antennas, conventional metallic conductors are associated with a fracture strain of a few percent and a patterned metal layer that can be easily stretched over various antennas.
To further investigate the applicability of a conventional metallic conductor incorporated with a stretchable antenna, researchers from the Pennsylvania State University recently published their findings on a stretchable microstrip antenna. The microstrip antennas contained a dielectric substrate that was sandwiched between a conductive patch and ground plane. The conductive material chosen for this study was copper (Cu) because of its excellent conductive capabilities and low cost.
This stretchable Cu patch serves as the radiating element in the antenna and creates the structured process through which the bottom mesh layer can stretch and flatten. The bottom layer of this sensor, which is also referred to as the ground plane, sits directly on top of the human subject’s skin and can easily be bent according to the movements of the skin.
These layers come together to form a two-dimensional (2D) meshed lattice structure with horseshoe units. When compressed and stretched, the top Cu patch layer creates a double arch that eventually flattens and stretches. This movement, which improves the overall flexibility of the sensor, also reduces RF fluctuations between the antenna’s state.
Furthermore, the bottom mesh layer prevents any radio signals from potentially interacting with the skin, improving the overall safety of the sensor while also ensuring its energy efficiency by preventing the loss of energy from occurring between the sensor and the human subject’s skin.
References and Further Reading
Liu, Y., Wang, H., Zhao, W., et al. (2018). Flexible, Stretchable Sensors for Wearable Health Monitoring: Sensing Mechanisms, Materials, Fabrication Strategies and Features. Sensors 18(2); 645. doi:10.3390/s18020645
Xu, G., Yuan, L., Chen, X., et al. (2021). Design of non-dimensional parameters in stretchable microstrip antennas with coupled mechanics-electromagnetics. Materials & Design 205. doi:10.1016/j.matdes.2021.109721
Penn State builds wearable antenna to transmit health monitoring data [Online]. Available from: https://www.beckershospitalreview.com/digital-transformation/penn-state-builds-wearable-antenna-to-transmit-health-monitoring-data.html
Stretching the boundaries of medical tech with wearable antennas [Online]. Available from: https://news.psu.edu/story/654780/2021/04/14/research/stretching-boundaries-medical-tech-wearable-antennas
Disclaimer: The views expressed here are those of the author expressed in their private capacity and do not necessarily represent the views of AZoM.com Limited T/A AZoNetwork the owner and operator of this website. This disclaimer forms part of the Terms and conditions of use of this website.